Types of PV modules: Mono & Poly
There are 3 types of PV modules that are currently existing: monocrystalline, polycrystalline and thin-film PV modules. However, the 2 most common types that dominate the market today are the monocrystalline and polycrystalline types, so these 2 will be the only focus of this article.
Method of Construction
The semiconductor material used in making monocrystalline PV modules are made by growing a single silicon crystal (hence, the name mono + crystalline). This means that the silicon has one crystal structure all throughout. Polycrystalline PV modules, on the other hand, are made by pouring molten silicon into a cast. This makes the crystal structure imperfect, having varying crystal structures all throughout the material. The difference in the crystal structures of the two are shown below:
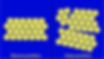
Since polycrystalline silicon has many crystal boundaries within the material, monocrystalline is theoretically more efficient because these boundaries impede the flow of electric current through it.
Which is Better, Monocrystalline or Polycrystalline?
Monocrystalline PV modules have the highest efficiency rates because they are made from the highest-grade silicon.
Since monocrystalline PV modules are more efficient, you will need less space to produce the same amount of power.
Monocrystalline PV modules have a lower temperature coefficient. With solar cells, power production decreases as the temperature increases. Having a lower temperature coefficient means that its power production is less affected by an increase in temperature.
Monocrystalline PV modules are more difficult to manufacture, and because of this, they are also more expensive.
Monocrystalline PV modules tend to be more aesthetically pleasing because of its uniform black color compared to the speckled blue color of polycrystalline ones.
Polycrystalline silicon is easier to manufacture and therefore, costs less. Also, the amount of waste silicon is also less compared to monocrystalline PV modules.
Although it seems from these comparisons that monocrystalline is better than polycrystalline PV modules, in reality, these differences are not that significant. In deciding which PV module to use in a project, there are other factors to consider like:
Brand and Model – different brands of PV modules offer products of varying qualities and features. It may be useful to look at Bloomberg’s Tier 1 PV modules list. Although this list is purely based on the “bankability” of the manufacturing company, it can also indirectly reflect on the quality of the PV modules that they offer to the public.
Price – different brands and models of PV modules have their own prices. It may sometimes be better to go for the worse module in terms of performance if the cost reduction is significant enough.
Availability and Logistics – this is something that is very important but is easily overlooked in the design process. A designer may spend hours and hours designing a solar PV system using one specific module, only to find out that that specific brand and model is not available. This works not only with a certain brand and model but also with the available PV module power ratings. You may design a system using 270W PV modules only to find out that the manufacturer or distributor only has 265W PV modules in stock.
Types of PV modules: 60-cells & 72-cells
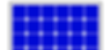
You may notice that the front of some PV modules is made up of little squares. These are the solar cells that are made from Silicon material. Each solar cell produces around 0.5 volts. These solar cells are wired in series inside the PV modules to raise the voltage to a useable level. PV module manufacturers most commonly use 60 cells and 72 cells in series per module.
60-cell PV modules are those that are in usually in the 210-280W range while the 72 cell PV modules are in the 300-340W range. 72-cell PV modules have the advantage of requiring fewer PV modules for a fixed system size. These 72 cell PV modules are still under the 50lb restriction by OSHA, which means that it is still considered safe to be carried by only one person. But its larger size may make it awkward to be carried by one person only.
Here are sample datasheets from Canadian Solar for their 60-cell and 72-cell PV modules:
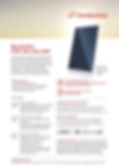
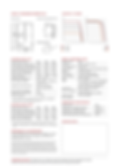

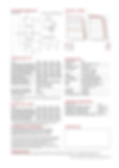
PV module Properties: IV Curve

The IV curve of Canadian Solar’s 255W polycrystalline PV modules is shown on the left.
The IV curve is the set of all possible operating points that the PV module may operate on, given a specific irradiance and temperature input. Specifically, it is the set of voltage and current pairs that the PV module may produce in its operation.
On the graph, the y-axis represents the current value while the x-axis represents the voltage value. The point where the curve intersects the x-axis is the VOC or the open-circuit voltage. The current value at this point is 0. The PV module operates on this point when the output is open-circuited. The point where the curve intersects the y-axis is the IsC or the short-circuit current. The voltage value at this point is 0 and the PV module operates on this point when the output is short-circuited.
As the irradiance input is increased, the IV curve goes “higher”. When this happens. the voltage values do not change much, but the current values change significantly. This represents the PV module characteristic of producing more power with a higher input irradiance.
The IV curve also changes with the module’s temperature. As the temperature rises, the current rises a little but the voltage decreases by a decent amount. This can be seen in the IV curve above for different temperatures. From here we can see how the other PV module characteristics change with temperature. Output current increases slightly with increases in temperature while the output voltage decreases.
PV module Properties: Power Curve & Maximum Power Point
Every point on the IV curve corresponds to a specific power output. This is shown in the graph below (blue curve). This is called the PV module’s power curve.

The point where the power production is at its maximum is called the MPP or Maximum Power Point. The voltage and current that correspond to this point are called the VMP and IMP, respectively. The PV module is most efficient when it operates at this point. In a PV array, the PV modules are “forced” by the inverter to operate at its maximum power point.
On a PV array, only one brand and model of the PV module is used. This is because different brands and models of PV modules have different MPPs and all PV modules on one inverter input can only operate on one voltage point. The inverter will have to choose a voltage point where the power produced by the whole PV array is at its maximum, even though it will cause the PV modules to not operate on their individual MPPs. This is illustrated by the image below:

The difference between the theoretical maximum power that the PV modules can produce (the sum of their power ratings) and the power produced in this scenario is called mismatch loss. Mismatch losses cannot be totally avoided even when using the same brand and model of PV modules because they may still have slight differences in their MPPs due to manufacturing imperfections.
PV module Properties: Efficiency
Commercially-available PV modules typically have efficiencies of 15-20%. This “low efficiency” of PV modules has been a point of attack by pro-nuclear and anti-renewable energy activists. The average efficiencies of the different types of power plants are shown below for comparison:

It is evident that solar PV has a relatively low efficiency compared to other types of power plants. But what does this mean? What does efficiency really mean? Efficiency is simply the ratio of energy output over the energy input expressed as a percentage. Therefore, comparing the efficiencies of the different types of power plants is like comparing apples and oranges because each of them has a different type of energy input.
The first law of thermodynamics states that energy can neither be created nor destroyed; it can only be transformed from one form to another. This is exactly what power plants do. They take energy in the form of a fuel and convert it into a useful form, which is electricity.
For fossil fuel and nuclear power plants, the input is in the form of various fuels. For solar, it is the light from the sun. You will already be able to see from here that comparing the efficiency of solar with these other energy sources is misleading. For fossil fuel power plants and nuclear, there are costs associated with the fuel, from mining to transportation and storage. With solar, the fuel is free, abundant and readily available. There are no additional resources needed to be expended to gather the fuel.
One way to make a fair comparison of these technologies is to compare their LCOEs or the Levelized Cost of Energies instead of the efficiency. The LCOE is the net present value of the unit-cost of electricity over the lifetime of a generating asset. You would have to sell all of the energy produced by your power plant over its lifetime at a price equal to the LCOE just to break even with all the costs associated with building, operating and maintaining the power plant over its lifetime. Or, stated more simply, if you are an investor and you will invest in building a certain power plant, selling the power that will be produced by your power plant at a price equal to the LCOE throughout its lifetime will just allow you to break even with your investment.

The graph on the previous page is from the 2017 edition of Lazard’s annual LCOE study. We can see here that currently, solar and wind already has a lower LCOE than all the other energy sources because of their continuously plummeting costs.
PV module Properties: Temperature Characteristic
The Thermal Characteristics part of the datasheet for Yingli’s YGE 235 Series PV modules is shown below:

There are three temperature coefficients defined here, one for power, and another for the voltage and currents. These values represent the percent change in power, voltage or current with an increase in solar cell temperature with respect to the standard operating cell temperature of 25 degrees Celsius.
Negative values for the temperature coefficients of power and voltage means that both of these parameters decrease with increasing temperature. Current, on the other hand, is a small positive number which means that it increases slightly with increases in temperature.
It is a common misconception about PV modules that it produces energy from heat. As we have learned in the article on the photoelectric effect, PV modules produce energy from sunlight instead. We also learned in this article that heat actually decreases the output of the PV modules.
It is also important to discuss here the difference between ambient temperature and cell temperature or operating temperature. Ambient temperature is the air temperature on the environment where the PV modules are installed. Cell temperature or operating temperature is the actual temperature of the solar cells inside the PV module while it is producing power. Ambient temperature is proportional to the cell temperature, but other factors also come into play like wind speed, the ventilation around the installed PV modules and the available irradiance. You can get a good approximation of the cell temperature by adding 25 degrees Celsius to the ambient temperature.
You will also notice on the datasheet that there are two sets of electrical parameters defined, one for Standard Test Conditions or STC and one for Nominal Operating Cell Temperature or NOCT. STC is defined at an irradiance of 1,000W/m2 and a cell temperature of 25 degrees Celsius. In real-life conditions, however, these values are rarely met simultaneously. A better approximation of real-life conditions is the NOCT, which is defined at an irradiance of 800W/m2 and an ambient temperature of 20 degrees Celsius.
Effect of Shading
Let us look at this scenario where we have a string of 5 PV modules connected with 1 of these PV modules shaded. There are 2 possible scenarios, one for slight shading and one for severe shading. Let us first consider the first scenario of slight shading.
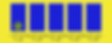
Having shade in one of the PV modules means that this module effectively receives less irradiance input. Because of this, its IV curve becomes lower. This causes it to produce a lower amount of current and voltage than the other PV modules in the string. The problem is that, since the PV modules are in series, they can only produce the same amount of current. The inverter’s MPPT function will always cause PV modules on a string to operate on the current and voltage values where the total power output of the string as a whole is at its maximum. In our example, the string as a whole will produce more power when the current value of the string is equal to the shaded module’s short circuit current because this is the closest possible current to the other PV module’s IMP. This effectively reduces the power output of all the other PV modules in the string. For a PV array, a small amount of shading can have a huge effect on overall system output as shown in this example and must be avoided as much as possible in the design.
Effect of Shading
Let us look at this scenario where we have a string of 5 PV modules connected with 1 of these PV modules shaded. There are 2 possible scenarios, one for slight shading and one for severe shading. Let us first consider the first scenario of slight shading.
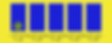
Having shade in one of the PV modules means that this module effectively receives less irradiance input. Because of this, its IV curve becomes lower. This causes it to produce a lower amount of current and voltage than the other PV modules in the string. The problem is that, since the PV modules are in series, they can only produce the same amount of current. The inverter’s MPPT function will always cause PV modules on a string to operate on the current and voltage values where the total power output of the string as a whole is at its maximum. In our example, the string as a whole will produce more power when the current value of the string is equal to the shaded module’s short circuit current because this is the closest possible current to the other PV module’s IMP. This effectively reduces the power output of all the other PV modules in the string. For a PV array, a small amount of shading can have a huge effect on overall system output as shown in this example and must be avoided as much as possible in the design.
For our second scenario of severe shading:
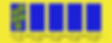
Similar to our first example, all PV modules can operate at a current equal to the shaded PV module’s ISC. However, this presents a significant decrease in the whole string’s overall power output. What will happen instead is that the unshaded PV modules will still operate at their maximum power current (IMP). Since this current is very far above the shaded PV module’s ISC, the only way that this could happen is if it operates on its negative voltage range. Operating in this region means that instead of the shaded PV module producing power, it will now consume power instead. This scenario is dangerous as it may cause damage to the shaded PV module.
Shading does not only affect the PV array at the string level but can also affect the solar cells inside the PV module in the same way. Remember that the solar cells are being connected in series inside the module to produces usable amounts of voltage. In the same way that severe shading on one PV module in a string can cause damage to that shaded PV module, shading one solar cell in the string of solar cells inside the PV module can also damage that shaded solar cell. This happens in the exact same way in the example above because solar cells basically have the same characteristics as PV modules. They also have the exact same IV curve, with the difference being that PV modules can produce higher voltages. When this happens on one solar cell inside a module, it is called hot spot heating. This may cause cell or glass cracking, melting of solder or degradation of the solar cell.
The destructive effects of hot spot heating can be avoided through the use of bypass diodes.
PV Module String Characteristics
We have already discussed the characteristics of strings of PV modules sparingly but it is just proper that we summarize all of those in a single place.
When PV modules are connected in series to form a string, their output voltages add up. The number of PV modules in a string is usually limited by the maximum array voltage which is usually at 1,000V DC. Because of this, available PV wires and DC disconnects for solar PV applications are usually rated at 1,000V DC.
PV modules connected in a string can only produce the same amount of current. This is the reason why if one module is shaded, all other modules in the string are affected. Also, because of this, PV modules in a string are always installed with a similar tilt and orientation for them to always produce the same amount of current. PV modules in a string, therefore, can be thought of as working as a single unit, with the whole string producing power only as efficient as the worst-performing PV module.
1,000V, 600V and 1,500V DC Solar/PV Array Systems
The first PV arrays have maximum string voltages of 600V because this is the standard low voltage limit in the National Electrical Code or NEC. 1,000V systems are then later proposed and implemented because of the savings associated with the reduced number of strings, wire lengths and required combiner boxes. Now, 1,500V systems are slowly gaining more popularity as this adds more savings from 1,000V systems. These added savings, however, are not as significant as when going from 600V systems to 1,000V.
Below is a table comparing three sample ground-mounted 100kW PV arrays designed using 600V, 1,000V and 1,500V as maximum string voltages:
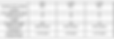
The number of PV modules per string increased by 67% from the 600V system to the 1,000V system and increased by 48% from the 1,000V system to the 1,500V system. This translated to a 41% decrease in the total number of strings from the 600V system to the 1,000V system and a 31% decrease from the 1,000V system to the 1,500V system.
Having more PV modules per string and a lower total number of strings have several advantages:
Less amount of required string wires - the 1,000V system required 58% less string wires compared to the 600V system and the 1,500V system required 23% less string wires compared to the 1,000V system.
Less amount or required MC4 connectors – MC4 connectors are used to extend the built-in PV wires on the PV modules. For every string on the system, you need an additional 4 of these (2 pairs).
Fewer and smaller combiner boxes – strings are usually combined with the use of combiner boxes to accommodate the limited inverter inputs. With fewer strings, fewer combiner boxes are required.
Easier and faster installation.
Lower currents which translate to lower losses.
However, having a higher maximum voltage also has some disadvantages. Installers will have to work with higher voltages and may need better protective equipment. Also, the balance of system components like wires, disconnects, etc. that are rated at a higher voltage, especially for 1,500V rated equipment, can cause problems in availability and procurement.
PV Module Physical Characteristics
PV modules are designed to withstand extreme weather conditions like variations in temperature, rain, snow, and hail for 25 years. This is also why in this book, we will only always recommend buying and using high-quality PV modules. Cheap PV modules that are manufactured just to be easily sold in the market are most likely to fail before their rated lifetime of 25 years. Worse, they may even cause fires, damaging property or even taking lives.
PV modules are usually made up of 6 components all of which are shown below:
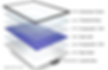
Solar Cells
Glass – the glass at the very front of the PV modules make them weatherproof and protects them from impact from falling debris. These are made from 3 to 4mm thick tempered glass. Tempered glass is used because it breaks only in tiny fragments rather than large, sharp and jagged sections for standard glass. The International Electrotechnical Commission (IEC) minimum standard is that the glass must be able to withstand an impact from 1-inch wide hailstones traveling at 60 miles per hour.
Aluminum Frame – the aluminum frame protects the edge of the laminate section containing the solar cells while also providing a solid structure to mount the solar cells. The frame is made from aluminum because of its lightweight characteristic. The PV modules are mounted in position by clamps installed on the frame. The frame is also grounded in the installation for protection.
EVA film – EVA (ethylene vinyl acetate) is a highly transparent plastic used to encapsulate the solar cells. It has 2 functions, as shock absorption from external impact and as another layer of protection from temperature and moisture and dirt ingress.
Back sheet – the back sheet is located on the rearmost part of the PV module for mechanical protection and electrical insulation.
Junction Box – the junction box is where all the solar cells interconnect. It also houses the external cables for interconnection with other PV modules. It is located near the top of the rear part of the PV module. It also houses the bypass diodes, which are used to prevent reverse currents into the solar cells due to shading.
Bypass Diodes
Hot spot heating can be avoided through the use of bypass diodes. But before we discuss bypass diodes, a quick introduction to diodes, in general, is in order.
Diodes are semiconductor devices that allow current to flow only in one direction. It has two terminals, the anode (+) and the cathode (-). When a positive voltage is applied to the anode with respect to the cathode, the diode is said to be forward-biased. In this case, it acts as a short-circuit and conducts current. When the reverse happens, or the cathode has a positive voltage with respect to the anode, the diode is said to be reverse-biased. It then acts as an open-circuit and thus, will not conduct current except for a small amount of reverse current. Bypass diodes also work exactly this way. Some PV modules have built-in bypass diodes already.
They are connected in parallel to the solar cells in series inside the PV modules. But since there are 60 or 72 solar cells in series inside the PV module it will be expensive and impractical to put a bypass diode in each. Thus, the number of bypass diodes typically used by PV module manufacturers range usually ranges from just 2-4 pieces.
Bypass diodes are connected in a way such that each is connected to an equal number of solar cells. For a 60-cell PV module with 4 bypass diodes, each bypass diode is connected in parallel to 15 solar cells each.
To illustrate how bypass diodes protect the solar cells from hot spot heating, let us say that we have a PV module with 8 solar cells (for simplicity) and 2 bypass diodes. The bypass diodes are connected in parallel with the solar cells in such a way that during normal operation (no shading), they are reverse biased.
This is what happens with the solar cell voltages with one shaded solar cell:
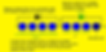
In the symbol for the diode on the image above, the left side of the arrow is the anode and the right side is the cathode. As we can see, the anode has a more positive voltage than the cathode. This will cause the bypass diode to be forward biased and thus, it will act as a short-circuit. Because of this, the current from the other good solar cells will flow through the bypass diode instead of the shaded solar cell preventing damage to it.
Engr. Jet Andal has 6 years of experience in the design and installation of residential, commercial and utility-scale solar PV systems. Together, and with the use of solar energy, let us help make the world a better place. You can click here to read all of our other blogs. For aspiring solar PV engineers, you can also check out his Solar PV Engineering Ebook on Amazon on this link.